In the 1850s, immigrants seeking gold in California’s Sierra Nevada mountains created a connected network of open channels, wooden flumes, and pipes to direct water to their operations and the rapidly-growing foothill towns of Sonora, Columbia, and Jamestown.
170 years later, this historic system is an integral part of the water infrastructure that supports residential, agricultural, hydroelectric, ecosystem, and recreational purposes, providing nearly all of the drinking water to the west slope communities of Tuolumne County. Tuolumne Utilities District (TUD), the agency responsible for managing the 70-plus miles of raw water ditches and potable water infrastructure, is contending with a modern-day concern: the threat of severe wildfire.
Conservation Biology Institute and EN2 Resources, Inc. (en2resources.com/) partnered to develop the TUD Wildfire Defense Plan, which was adopted by Tuolumne Utilities District (TUD) in April of 2025.
This comprehensive strategy to protect Tuolumne County’s water resources and increase the community’s resilience to wildfire aligns with and implements the Tuolumne County Community Wildfire Protection Plan (CWPP). This plan also follows state and federal government directives relating to wildfire resilience for California’s communities and forests, including the California Wildfire and Forest Resilience Task Force’s Wildfire and Forest Resilience Action Plan and the U.S. Forest Service’s Wildfire Crisis Strategy Implementation Plan.
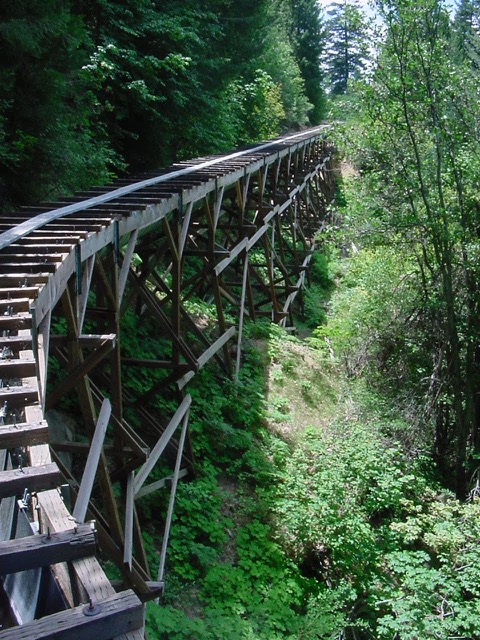
The TUD Wildfire Defense Plan contains two components:
TUD Wildfire Risk Mitigation Plan, prepared by CBI in consultation with TUD, presents prioritized locations for mitigating wildfire risks to TUD infrastructure and supports the effective planning and implementation of the prioritized projects while providing co-benefits to the community and natural resources.
The TUD Fire Water Draft Points Plan, prepared by EN2 Resources in consultation with TUD, identifies 49 strategic raw water draft points across the district to increase water availability for fire suppression, reduce demands on potable water systems, and enhance emergency response capabilities.Together these plans will help TUD manage the system as “green infrastructure”, a vision that addresses the integrated needs of people, the communities, and biodiversity under climate change. TUD, together with Pacific Gas & Electric, the US Forest Service, CAL FIRE, Tuolumne County, Tuolumne Fire Safe Council and the Tuolumne Band of Me-Wuk Indians are already working intensively to reduce fuels in the region, and this Plan will assist the agency in obtaining the funding needed to continue this important work.
The TUD Wildfire Defense Plan was developed with funding from the USDA Forest Service Community Wildfire Defense Grant. For more information about the TUD Wildfire Defense Plan, please visit https://tudwater.com/wildfire-defense-plan/.
Abstract: Wildfires can be devastating for social and ecological systems, but the recovery period after wildfire presents opportunities to reduce future risk through adaptation. We use a collective case study approach to systematically compare social and ecological recovery following four major fire events in Australia and the United States: the 1998 wildfires in northeastern Florida; the 2003 Cedar fire in southern California; the 2009 Black Saturday bushfires in Victoria, southeastern Australia; and the 2011 Bastrop fires in Texas. Fires spurred similar policy changes, with an emphasis on education, land use planning, suppression/emergency response, and vegetation management. However, there was little information available in peer-reviewed literature about social recovery, ecological recovery was mostly studied short term, and feedbacks between social and ecological outcomes went largely unconsidered. Strategic and holistic approaches to wildfire recovery that consider linkages within and between social–ecological systems will be increasingly critical to determine if recovery leads to adaptation or recreates vulnerability.
The severity and frequency of wildfires have increased throughout the Pacific Northwest in recent decades, costing lives and destroying large amounts of valuable resources and assets. This trend is predicted to persist because of climate change and the associated increased fire risk caused by prolonged droughts in combination with changes in land cover and land use, including rapid increases in wildland urban interface areas. The threat of benzene and other contaminants in drinking water from water distribution systems after wildfires is a relatively recently discovered problem that gained attention because of the significant health hazards that high levels of benzene in drinking water pose for humans. Driving processes leading to post-fire benzene contamination in water distribution systems are largely unknown. Currently, no deterministic process models exist to predict the risk of exceeded benzene levels in water distribution systems after wildfires. To address the lack of predictive models, we developed and tested an approach based on neural network models to spatially predict the conditional probabilities of exceeding maximum contaminant levels for benzene after wildfires. The Bayesian regularized neural networks were trained using high-resolution data layers comprising topography, soil properties, landcover, vegetation, meteorological parameters, fuel load, and infrastructure data for two wildland urban interface areas in northern California. The generalized model ensemble encompassing data from both communities exhibits an accuracy of 83% to 88% in spatially predicting the post-fire exceedance of benzene levels, offering a planning tool for emergency response and future risk mitigation efforts.\r\
One consequence of global change causing widespread concern is the possibility of ecosystem conversions from one type to another. A classic example of this is vegetation type conversion (VTC) from native woody shrublands to invasive annual grasslands in the biodiversity hotspot of Southern California. Although the significance of this problem is well recognized, understanding where, how much, and why this change is occurring remains elusive owing to differences in results from studies conducted using different methods, spatial extents, and scales. Disagreement has arisen particularly over the relative importance of short-interval fires in driving these changes. Chronosequence approaches that use space for time to estimate changes have produced different results than studies of changes at a site over time. Here we calculated the percentage woody and herbaceous cover across Southern California using air photos from ~1950 to 2019. We assessed the extent of woody cover change and the relative importance of fire history, topography, soil moisture, and distance to human infrastructure in explaining change across a hierarchy of spatial extents and regions. We found substantial net decline in woody cover and expansion of herbaceous vegetation across all regions, but the most dramatic changes occurred in the northern interior and southern coastal areas. Variables related to frequent, short-interval fire were consistently top ranked as the explanation for shrub to grassland type conversion, but low soil moisture and topographic complexity were also strong correlates. Despite the consistent importance of fire, there was substantial geographical variation in the relative importance of drivers, and these differences resulted in different mapped predictions of VTC. This geographical variation is important to recognize for management decision-making and, in addition to differences in methodological design, may also partly explain differences in previous study results. The overwhelming importance of short-interval fire has management implications. It suggests that actions should be directed away from imposing fires to preventing fires. Prevention can be controlled through management actions that limit ignitions, fire spread, and the damage sustained in areas that do burn. This study also demonstrates significant potential for changing fire regimes to drive large-scale, abrupt ecological change.
Recent increases in destructive wildfires are driving a need for empirical research documenting factors that contribute to structure loss. Existing studies show that fire risk is complex and varies geographically, and the role of vegetation has been especially difficult to quantify. Here, we evaluated the relative importance of vegetation cover at local (measured through the Normalized Difference Vegetation Index) and landscape (as measured through the Wildland–Urban Interface) scales in explaining structure loss from 2013 to 2018 in California—statewide and divided across three regions. Generally, the pattern of housing relative to vegetation better explained structure loss than local-scale vegetation amount, but the results varied regionally. This is likely because exposure to fire is a necessary first condition for structure survival, and sensitivity is only relevant once the fire reaches there. The relative importance of other factors such as long-term climatic variability, distance to powerlines, and elevation also varied among regions. These suggest that effective fire risk reduction strategies may need to account for multiple factors at multiple scales. The geographical variability in results also reinforces the notion that “one size does not fit all”. Local-scale empirical research on specific vegetation characteristics relative to structure loss is needed to inform the most effective customized plan.
California has earned a reputation for wildfires that inflict serious damage on human infrastructure, dating back to images of Richard Nixon hosing down the roof of his house in the 1961 Bel-Air fire, and of the famous “fireproof” home of grocery store entrepreneur Fred Roberts burning to the ground in 1982. In recent years, this notoriety has been transformed into public alarm, reflected in the apocalyptic headlines of recent newspaper articles suggesting the “end of California” (New York Times, 30 October 2019) and that “California is becoming unlivable” (The Atlantic, 30 October 2019). Now the phrase “the new normal” has worked its way into the lexicon, sustained by record-breaking struc- ture loss numbers in 2017 and 2018 despite significantly lower structure losses in 2019.
It remains to be seen whether or not those two recent years were back-to-back one-in-a-hundred-year events, or if the trend has crossed some kind of tipping point, but data do show a longterm trend of significant increase in structures lost to wildfires since the beginning of the 20th century (Fig. 1). What was an average of ~500 homes lost per year in Southern California from about 1950–2000 (CalFire 2000) has recently climbed to ~2700 structures per year statewide from 2000–2018 (Syphard and Keeley 2019). California is not alone in the U.S., or in the world, in suffering increasing impacts from wildfires (e.g., Blanchi et al. 2012, Haynes 2015, Viegas 2018). Impacts so far in the current Australian bushfire season have been recordbreaking, with several thousand structures lost, more than 25 fatalities, and unthinkable losses to wildlife. The question that follows, then, is why?
Fire has been a source of global biodiversity for millions of years. However, interactions with anthropogenic drivers such as climate change, land use, and invasive species are changing the nature of fire activity and its impacts. We review how such changes are threatening species with extinction and transforming terrestrial ecosystems. Conservation of Earth’s biological diversity will be achieved only by recognizing and responding to the critical role of fire. In the Anthropocene, this requires that conservation planning explicitly includes the combined effects of human activities and fire regimes. Improved forecasts for biodiversity must also integrate the connections among people, fire, and ecosystems. Such integration provides an opportunity for new actions that could revolutionize how society sustains biodiversity in a time of changing fire activity.
The climate emergency has arrived and is accelerating more rapidly than most scientists anticipated, and many of them are deeply concerned. The adverse effects of climate change are much more severe than expected, and now threaten both the biosphere and humanity. There is mounting evidence linking increases in extreme weather frequency and intensity to climate change. The year 2020, one of the hottest years on record, also saw extraordinary wildfire activity in the Western United States and Australia, a Siberian heat wave with record high temperatures exceeding 38 degrees C (100.4 degrees Fahrenheit) within the Arctic circle, a record low for October Arctic sea ice extent of 2.04 million square miles, an Atlantic hurricane season resulting in more than $46 billion in damage, and deadly floods and landslides in South Asia that displaced more than 12 million people.
The important role of fire in regulating vegetation community composition and contributions to emissions of greenhouse gases and aerosols make it a critical component of dynamic global vegetation models and Earth system models. Over two decades of development, a wide variety of model structures and mechanisms have been designed and incorporated into global fire models, which have been linked to different vegetation models. However, there has not yet been a systematic examination of how these different strategies contribute to model performance. Here we describe the structure of the first phase of the Fire Model Intercomparison Project (FireMIP), which for the first time seeks to systematically compare a number of models. By combining a standardized set of input data and model experiments with a rigorous comparison of model outputs to each other 5 and to observations, we will improve the understanding of what drives vegetation fire, how it can best be simulated, and what new or improved observational data could allow better constraints on model behavior. Here we introduce the fire models used in the first phase of FireMIP, the simulation protocols applied, and the benchmarking system used to evaluate the models.
The relationship between annual variation in area burned and seasonal temperatures and precipitation was investigated for the major climate divisions in California. Historical analyses showed marked differences in fires on montane and foothill landscapes. Based on roughly a century of data, there are five important lessons on fire–climate relationships in California: (1) seasonal variations in temperature appear to have had minimal influence on area burned in the lower elevation, mostly non-forested, landscapes; (2) temperature has been a significant factor in controlling fire activity in higher elevation montane forests, but this varied greatly with season – winter and autumn temperatures showed no significant effect, whereas spring and summer temperatures were important determinants of area burned; (3) current season precipitation has been a strong controller of fire activity in forests, with drier years resulting in greater area burned on most United States Forest Service (USFS) lands in the state, but the effect of current-year precipitation was decidedly less on lower elevation California Department of Forestry and Fire Protection lands; (4) in largely grass-dominated foothills and valleys the magnitude of prior-year rainfall was positively tied to area burned in the following year, and we hypothesise that this is tied to greater fuel volume in the year following high rainfall. In the southern part of the state this effect has become stronger in recent decades and this likely is due to accelerated type conversion from shrubland to grassland in the latter part of the 20th century; (5) the strongest fire–climate models were on USFS lands in the Sierra Nevada Mountains, and these explained 42–52% of the variation in area burned; however, the models changed over time, with winter and spring precipitation being the primary drivers in the first half of the 20th century, but replaced by spring and summer temperatures after 1960.